Monomeric, porous type II collagen scaffolds promote chondrogenic differentiation of human bone marrow mesenchymal stem cells in vitro
- PMID: 28256634
- PMCID: PMC5335259
- DOI: 10.1038/srep43519
Monomeric, porous type II collagen scaffolds promote chondrogenic differentiation of human bone marrow mesenchymal stem cells in vitro
Abstract
Osteoarthritis (OA) is a common cause of pain and disability and is often associated with the degeneration of articular cartilage. Lesions to the articular surface, which are thought to progress to OA, have the potential to be repaired using tissue engineering strategies; however, it remains challenging to instruct cell differentiation within a scaffold to produce tissue with appropriate structural, chemical and mechanical properties. We aimed to address this by driving progenitor cells to adopt a chondrogenic phenotype through the tailoring of scaffold composition and physical properties. Monomeric type-I and type-II collagen scaffolds, which avoid potential immunogenicity associated with fibrillar collagens, were fabricated with and without chondroitin sulfate (CS) and their ability to stimulate the chondrogenic differentiation of human bone marrow-derived mesenchymal stem cells was assessed. Immunohistochemical analyses showed that cells produced abundant collagen type-II on type-II scaffolds and collagen type-I on type-I scaffolds. Gene expression analyses indicated that the addition of CS - which was released from scaffolds quickly - significantly upregulated expression of type II collagen, compared to type-I and pure type-II scaffolds. We conclude that collagen type-II and CS can be used to promote a more chondrogenic phenotype in the absence of growth factors, potentially providing an eventual therapy to prevent OA.
Conflict of interest statement
The authors declare no competing financial interests.
Figures


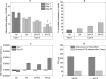


Similar articles
-
Hypoxic culture of bone marrow-derived mesenchymal stromal stem cells differentially enhances in vitro chondrogenesis within cell-seeded collagen and hyaluronic acid porous scaffolds.Stem Cell Res Ther. 2015 Apr 23;6(1):84. doi: 10.1186/s13287-015-0075-4. Stem Cell Res Ther. 2015. PMID: 25900045 Free PMC article.
-
Similar properties of chondrocytes from osteoarthritis joints and mesenchymal stem cells from healthy donors for tissue engineering of articular cartilage.PLoS One. 2013 May 9;8(5):e62994. doi: 10.1371/journal.pone.0062994. Print 2013. PLoS One. 2013. PMID: 23671648 Free PMC article.
-
Chondrogenesis of human bone marrow mesenchymal stromal cells in highly porous alginate-foams supplemented with chondroitin sulfate.Mater Sci Eng C Mater Biol Appl. 2015 May;50:160-72. doi: 10.1016/j.msec.2015.01.082. Epub 2015 Jan 27. Mater Sci Eng C Mater Biol Appl. 2015. PMID: 25746258
-
Chondrogenic differentiation of synovial fluid mesenchymal stem cells on human meniscus-derived decellularized matrix requires exogenous growth factors.Acta Biomater. 2018 Oct 15;80:131-143. doi: 10.1016/j.actbio.2018.09.038. Epub 2018 Sep 26. Acta Biomater. 2018. PMID: 30267878
-
In vitro study of cartilage tissue engineering using human adipose-derived stem cells induced by platelet-rich plasma and cultured on silk fibroin scaffold.Stem Cell Res Ther. 2019 Dec 4;10(1):369. doi: 10.1186/s13287-019-1443-2. Stem Cell Res Ther. 2019. PMID: 31801639 Free PMC article.
Cited by 21 articles
-
Targeted mesenchymal stem cell therapy equipped with a cell-tissue nanomatchmaker attenuates osteoarthritis progression.Sci Rep. 2022 Mar 7;12(1):4015. doi: 10.1038/s41598-022-07969-9. Sci Rep. 2022. PMID: 35256711 Free PMC article.
-
Layer-specific stem cell differentiation in tri-layered tissue engineering biomaterials: Towards development of a single-stage cell-based approach for osteochondral defect repair.Mater Today Bio. 2021 Nov 27;12:100173. doi: 10.1016/j.mtbio.2021.100173. eCollection 2021 Sep. Mater Today Bio. 2021. PMID: 34901823 Free PMC article.
-
Methods of Modification of Mesenchymal Stem Cells and Conditions of Their Culturing for Hyaline Cartilage Tissue Engineering.Biomedicines. 2021 Nov 11;9(11):1666. doi: 10.3390/biomedicines9111666. Biomedicines. 2021. PMID: 34829895 Free PMC article. Review.
-
Host Response to Biomaterials for Cartilage Tissue Engineering: Key to Remodeling.Front Bioeng Biotechnol. 2021 May 4;9:664592. doi: 10.3389/fbioe.2021.664592. eCollection 2021. Front Bioeng Biotechnol. 2021. PMID: 34017827 Free PMC article. Review.
-
Nanofibrous Gelatin-Based Biomaterial with Improved Biomimicry Using D-Periodic Self-Assembled Atelocollagen.Biomimetics (Basel). 2021 Mar 18;6(1):20. doi: 10.3390/biomimetics6010020. Biomimetics (Basel). 2021. PMID: 33803778 Free PMC article.
References
-
- Buckwalter J. A. & Mankin H. J. Articular cartilage: degeneration and osteoarthritis, repair, regeneration, and transplantation. Instr. Course Lect. 47, 487–504 (1998). - PubMed
-
- Newman A. P. Articular cartilage repair. Am. J. Sports Med. 26, 309–324 (1998). - PubMed
-
- Lynn A. K., Brooks R. A., Bonfield W. & Rushton N. Repair of defects in articular joints. Prospects for material-based solutions in tissue engineering. J. Bone Joint Surg. Br. 86B, 1093–1099 (2004). - PubMed
Publication types
MeSH terms
Substances
Grant support
LinkOut - more resources
Full Text Sources
Other Literature Sources