Long Noncoding RNA GAS5 Contained in Exosomes Derived from Human Adipose Stem Cells Promotes Repair and Modulates Inflammation in a Chronic Dermal Wound Healing Model
- PMID: 35336800
- PMCID: PMC8945809
- DOI: 10.3390/biology11030426
Long Noncoding RNA GAS5 Contained in Exosomes Derived from Human Adipose Stem Cells Promotes Repair and Modulates Inflammation in a Chronic Dermal Wound Healing Model
Abstract
Chronic recalcitrant wounds result from delayed or slowed healing processes. Underlying inflammation is a substantial risk factor for impaired dermal wound healing and often leads to chronic wound-related sequelae. Human adipose stem cells (hASCs) have shown tremendous potential in regenerative medicine. The goal of this project was to improve the outcome of chronic wounds by harvesting the exosomes from hASCs for therapeutic intervention. The results demonstrate that long noncoding RNA GAS5 is highly enriched in hASC exosomes and, further, that GAS5 is central to promoting wound repair in vitro. To evaluate the outcome of wound healing in a chronic low-grade inflammatory environment, lipopolysaccharide-treated HDF cells were evaluated for their response to hASC exosome treatment. Ingenuity pathway analysis identified inflammation pathways and genes affected by exosomes in a GAS5-dependent manner. Using siRNA to deplete GAS5 in HDF, the results demonstrated that Toll-like receptor 7 (TLR7) expression levels were regulated by GAS5. Importantly, the results demonstrate that GAS5 regulates inflammatory pathway genes in a chronic inflammation environment. The results presented here demonstrate that hASC exosomes are a viable therapeutic that accelerate the healing of chronic recalcitrant wounds.
Keywords: GAS5; chronic wounds; exosomes; human adipose stem cells; human dermal fibroblast (HDF); inflammation; lipopolysaccharide (LPS); long noncoding RNA (lncRNA); wound healing.
Conflict of interest statement
The authors declare no conflict of interest with regard to this manuscript.
Figures

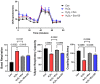



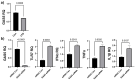
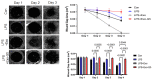
References
-
- Patel R.S., Carter G., El Bassit G., Patel A.A., Cooper D.R., Murr M., Patel N.A. Adipose-derived stem cells from lean and obese humans show depot specific differences in their stem cell markers, exosome contents and senescence: Role of protein kinase C delta (PKCdelta) in adipose stem cell niche. Stem Cell Investig. 2016;3:2. - PMC - PubMed